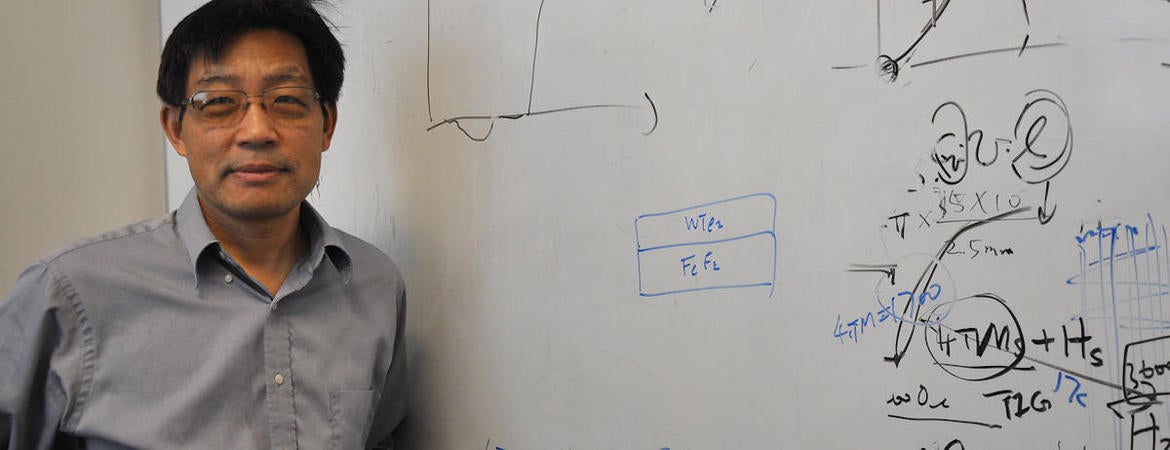
A team of physicists has discovered an electrical detection method for terahertz electromagnetic waves, which are extremely difficult to detect. The discovery could help miniaturize the detection equipment on microchips and enhance sensitivity.
Terahertz is a unit of electromagnetic wave frequency: One gigahertz equals 1 billion hertz; 1 terahertz equals 1,000 gigahertz. The higher the frequency, the faster the transmission of information. Cell phones, for example, operate at a few gigahertz.
The finding, reported today in Nature, is based on a magnetic resonance phenomenon in anti-ferromagnetic materials. Such materials, also called antiferromagnets, offer unique advantages for ultrafast and spin-based nanoscale device applications.
The researchers, led by physicist Jing Shi of the University of California, Riverside, generated a spin current, an important physical quantity in spintronics, in an antiferromagnet and were able to detect it electrically. To accomplish this feat, they used terahertz radiation to pump up magnetic resonance in chromia to facilitate its detection.
In ferromagnets, such as a bar magnet, electron spins point in the same direction, up or down, thus providing collective strength to the materials. In antiferromagnets, the atomic arrangement is such that the electron spins cancel each other out, with half of the spins pointing in the opposite direction of the other half, either up or down.
The electron has a built-in spin angular momentum, which can precess the way a spinning top precesses around a vertical axis. When the precession frequency of electrons matches the frequency of electromagnetic waves generated by an external source acting on the electrons, magnetic resonance occurs and is manifested in the form of a greatly enhanced signal that is easier to detect.
In order to generate such magnetic resonance, the team of physicists from UC Riverside and UC Santa Barbara worked with 0.24 terahertz of radiation produced at the Institute for Terahertz Science and Technology’s Terahertz Facilities at the Santa Barbara campus. This closely matched the precession frequency of electrons in chromia. The magnetic resonance that followed resulted in the generation of a spin current that the researchers converted into a DC voltage.
“We were able to demonstrate that antiferromagnetic resonance can produce an electrical voltage, a spintronic effect that has never been experimentally done before,” said Shi, a professor in the Department of Physics and Astronomy.
Shi, who directs Department of Energy-funded Energy Frontier Research Center Spins and Heat in Nanoscale Electronic Systems, or SHINES, at UC Riverside, explained subterahertz and terahertz radiation are a challenge to detect. Current communication technology uses gigahertz microwaves.
“For higher bandwidth, however, the trend is to move toward terahertz microwaves,” Shi said. “The generation of terahertz microwaves is not difficult, but their detection is. Our work has now provided a new pathway for terahertz detection on a chip.”
Although antiferromagnets are statically uninteresting, they are dynamically interesting. Electron spin precession in antiferromagnets is much faster than in ferromagnets, resulting in frequencies that are two-three orders of magnitude higher than the frequencies of ferromagnets — thus allowing faster information transmission.
“Spin dynamics in antiferromagnets occur at a much shorter timescale than in ferromagnets, which offers attractive benefits for potential ultrafast device applications,” Shi said.
Antiferromagnets are ubiquitous and more abundant than ferromagnets. Many ferromagnets, such as iron and cobalt, become antiferromagnetic when oxidized. Many antiferromagnets are good insulators with low dissipation of energy. Shi’s lab has expertise in making ferromagnetic and antiferromagnetic insulators.
Shi’s team developed a bilayer structure comprised of chromia, an antiferromagnetic insulator, with a layer of metal on top of it to serve as the detector to sense signals from chromia.
Shi explained that electrons in chromia remain local. What crosses the interface is information encoded in the precessing spins of the electrons.
“The interface is critical,” he said. “So is spin sensitivity.”
The researchers addressed spin sensitivity by focusing on platinum and tantalum as metal detectors. If the signal from chromia originates in spin, platinum and tantalum register the signal with opposite polarity. If the signal is caused by heating, however, both metals register the signal with identical polarity.
“This is the first successful generation and detection of pure spin currents in antiferromagnetic materials, which is a hot topic in spintronics,” Shi said. “Antiferromagnetic spintronics is a major focus of SHINES.”
The technology has been disclosed to UCR Technology Commercialization, assigned UC case number 2019-105, and is patent pending.
Shi was joined in the study by Junxue Li, Ran Cheng, Mark Lohmann, Wei Yuan, Mohammed Aldosary, and Peng Wei of UC Riverside; and C. Blake Wilson, Marzieh Kavand, Nikolay Agladze, and Mark S. Sherwin at UC Santa Barbara.
The research at UC Riverside was supported by SHINES.
Header image: Photo shows Jing Shi, a professor of physics and astronomy at UC Riverside. (UCR/I. Pittalwala)